Are derivations of physical laws less important than the laws themselves?
Not necessarily. The thing to remember is that "physical laws" do not actually exist. What exists is space and light and matter and fields and other things too. These real things behave the way that they do because of the way that they are. We describe their behaviour using physical laws, and derivations of those physical laws. But neither are real things that really exist. And you can't be sure that that some accident of history means the derivation ought to have been the law and vice versa. Either way, the one that's most important is arguably the one that delivers insight and scientific progress in understanding those real things.
The proportionality between the kinetic energy of gas molecules and temperature is a well-known result.
Because temperature is an emergent property of motion. What's real is the container, and the gas, which is made up of molecules, which are moving. $\ P = \frac{2}{3}\frac{N}{V}\overline{KE}$ is merely a mathematical expression that relates a variety of measurements. Ditto for $PV = NRT$. The number of terms in the expression doesn't necessarily mean it's more important than some other expression.
GNUFDL image by Greg L see Wikipedia
This is usually shown by considering a cubical box containing an ideal gas, and postulating that the molecules obey Newton's Laws. After a few steps of quite intuitive justifications, we arrive at $\ P = \frac{2}{3}\frac{N}{V}\overline{KE}$, and along with the empirical ideal gas law, $PV = NRT$, we get our desired conclusion.
And an understanding of what the ideal gas law is describing. A container of gas molecules, bouncing off the walls, exerting pressure on those walls. The more massive they are, the more they are, and the faster they are, the greater the pressure.
But this seems too good to be true. We only considered a cubical box. Will the result still hold if we take an arbitrary box, with an arbitrary shape?
I think that's the wrong approach myself. How on earth would you draw up a law or come up with some derivation if you plump for the most complicated scenario possible? Instead you opt for the simplest scenario possible to reduce the complexity of the issue.
A student who has taken a fairly advanced Statistical Mechanics course will say yes, there is a more general and rigorous argument that reproduces our much loved $\ P = \frac{2}{3}\frac{N}{V}\overline{KE}$, using integrals and so on. However, an experienced physicist will shrug off this question and claim that it doesn't matter how you got to the result, because the proportionality of temperature and kinetic energy of an ideal gas is a well-established experimental fact.
I think he's more likely to shrug it off because he understands why that proportionality is there, and that all the expression is saying is that one thing you can measure relates to another because they're different ways of measuring what happens when molecules rattle around inside a box.
As much as I'd want to accept this explanation, I can't. I'll try to explain why. In astrophysics, there's the Schwarzschild radius, given by $\ R_{s}= \frac{2GM}{c^2}$. What's weird about this result is that it requires General Relativity, but there's a classical, but dubious derivation, using the escape velocity formula, $v_{escape} = \sqrt{\frac{2GM}{R}}$. A physicist will claim that this derivation is wrong because Newtonian Mechanics breaks down near or at light-speed, and the result is just a benign coincidence.
One physicist might say it's dubious because it isn't in accord with Einstein's general relativity:
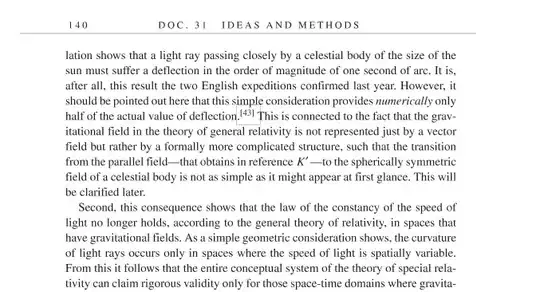
Another might say it works because a body falls because of the speed of light is spatially variable, and that the speed of a falling body is related to the spatial variability in the speed of light. And another might say it's misleading. There's always going to be disagreements. Life would be dull if we all agreed about everything.
However, this remark is very different from the attitude taken earlier, that we only need to conduct observations to validate a result, and it doesn't really matter how we arrived at it. Going back to the earlier ideal gas result, how do we know that the argument using a cubical box is not just a happy coincidence?
Because we understand the physics. We understand those real things and the way they are.
I am very frustrated that sometimes, physicists will be satisfied with a completely heuristic thought experiment to justify a result, and at other times, papers and books are written meticulously in the definition-theorem-proof style. For example, Schoen and Yau's papers on the Positive Energy Theorem, and Hawking and Ellis' book, The Large Scale Structure of Spacetime, which is considered a standard in its field, written in painstaking mathematical detail.
I think you're right to have concerns. I'm not sure I think your concerns are the right concerns.
Furthermore, I've read a lot of papers published in reputable journals where theoreticians attempt to provide more general and rigorous "proofs" of well-known results. Penrose's derivation of $E = mc^2$ (1965) is a case in point. Why bother doing this?
To attempt to assert himself. IMHO Penrose has a habit of appealing to Einstein's authority whilst flatly contradicting the guy and then warbling on about fantasy physics such as the parallel antiverse.
I myself am more satisfied if the results can be obtained rigorously. But as a student, I'm paying a high-price, because I don't seem to be progressing at an acceptable pace, owing to the fact that I spend too much time puzzling over the details of a physical derivation.
My advice is to try to "get under the maths" by focussing on what the terms actually mean. Then you'll understand those real things and the way they are. DOn't be distracted by abstract things that do not exist.